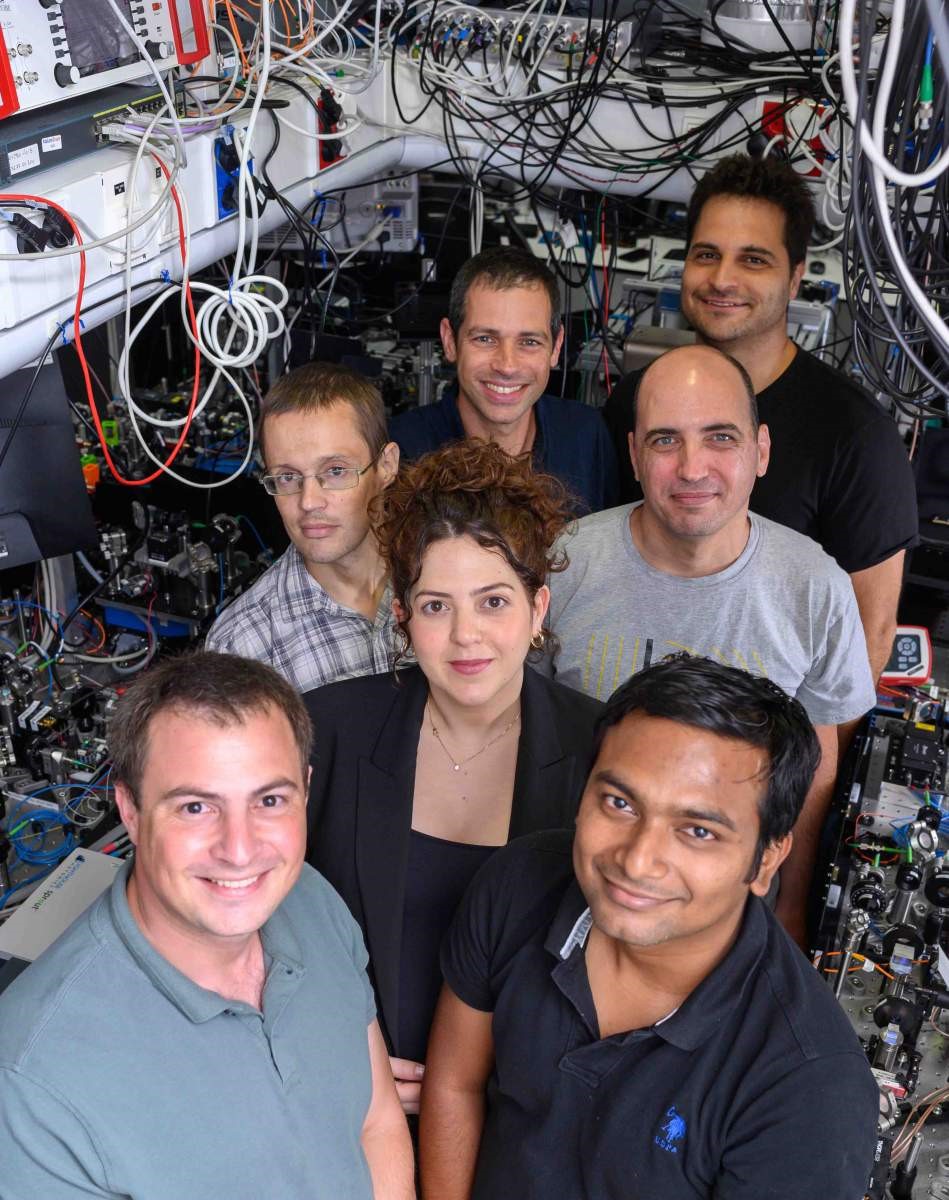
June 10, 2024
A Weizmann Institute study of photons in quantum computing has made a surprising discovery: When photons collide, they create vortices.
Vortices are a common physical phenomenon. You find them in the structure of galaxies, tornadoes and hurricanes, as well as in a cup of tea, or water as it drains from the bathtub. Normally, vortices are formed when very fast-flowing air, water or another substance encounters an area with a slower flow. They are characterised by circular flow around a stationary axis. The role of vortices, therefore, is to bridge the tension between adjacent areas with different speed flows.
A previously unknown type of vortex has now been discovered in a study, published in Science, conducted by Dr Lee Drori, Dr Bankim Chandra Das, Tomer Danino Zohar and Dr Gal Winer from Professor Ofer Firstenberg’s laboratory at the Weizmann Institute of Science’s Physics of Complex Systems Department.
The researchers set out to look for an efficient way of using photons to process data in quantum computers and found something unexpected: They realised that in the rare event that two photons interact, they create vortices.
Not only does this discovery add to the fundamental understanding of vortices, it may ultimately contribute to the study’s original goal of improving data processing in quantum computing.
The interaction between photons – light particles that also behave like waves – is only possible in the presence of matter that serves as an intermediary. In their experiment, the researchers forced photons to interact by creating a unique environment: a 10-centimeter glass cell that was completely empty, save for rubidium atoms that were so tightly packed in the centre of the container that they formed a small, dense gas cloud about 1 millimetre long. The researchers fired more and more photons through this cloud, examined their state after they had passed through it, and looked to see if they had influenced one another in any way.
“When the photons pass through the dense gas cloud, they send a number of atoms into electronically excited states known as Rydberg states,” Firstenberg explained.
“In these states, one of the electrons in the atom starts moving in an orbit that is 1,000 times wider than the diameter of an unexcited atom. This electron creates an electric field that influences a huge number of adjacent atoms, turning them into a kind of imaginary ‘glass ball’.”
The image of a glass ball reflects the fact that the second photon present in the area cannot ignore the environment the first photon has created and, in response, it alters its speed – as if it has passed through glass. So, when two photons pass relatively close to each other, they move at a different speed than they would have if each had been traveling alone. And when the speed of the photon changes, so does the position of the peaks and valleys of the wave it carries. In the optimal case for the use of photons in quantum computing, the positions of the peaks and valleys become completely inverted relative to one another, owing to the influence the photons have on each other – a phenomenon known as a 180-degree phase shift.
The direction that the research took was as unique and extraordinary as the paths of the photons in the gas cloud.
The study, which also included Dr Eilon Poem and Dr Alexander Poddubny, began eight years ago and has seen two generations of doctoral students pass through Firstenberg’s laboratory. Over time, the Weizmann scientists managed to create a dense, ultracold gas cloud, packed with atoms. As a result, they achieved something unprecedented: photons that underwent a phase shift of 180-degrees – and sometimes more. When the gas cloud was at its densest and the photons were close to each other, they exerted the highest level of mutual influence. But when the photons moved away from each other or the atomic density around them dropped, the phase shift weakened and disappeared.
The prevalent assumption was that this weakening would be a gradual process, but researchers were in for a surprise: A pair of vortices developed when two photons were a certain distance apart. In each of these vortices, the photons completed a 360-degree phase shift and, at their centre there were almost no photons at all – just as in the dark centre we know from other vortices.
To understand photon vortices, think of what happens when you drag a vertically held plate through the water. The rapid movement of the water pushed by the plate meets the slower movement around it. This creates two vortices that, when viewed from above, appear to be moving together along the water’s surface, but in fact, they are part of a three-dimensional configuration known as a vortex ring: The submerged part of the plate creates half a ring, which connects the two vortices visible on the surface, forcing them to move together. Another familiar instance of vortex rings is smoke rings. In the last stages of the study, the researchers observed this phenomenon when they introduced a third photon, which added an extra dimension to the findings: The scientists discovered that the two vortices observed when measuring two photons are part of a three-dimensional vortex ring generated by the mutual influence of the three photons. These findings demonstrate just how similar the newly discovered vortices are to those known from other environments.
The vortices may have stolen the show in this study, but the researchers are continuing to work toward their goal of quantum data processing. The next stage of the study will be to fire the photons into each other and measure the phase shift of each photon separately. Depending on the strength of the phase shifts, the photons could be used as qubits – the basic units of information in quantum computing. Unlike the units of regular computer memory, which can either be 0 or 1, quantum bits can represent a range of values between 0 and 1 simultaneously.
Professor Ofer Firstenberg’s research is supported by the Leona M. and Harry B. Helmsley Charitable Trust, the Shimon and Golde Picker – Weizmann Annual Grant and the Laboratory in Memory of Leon and Blacky Broder, Switzerland.
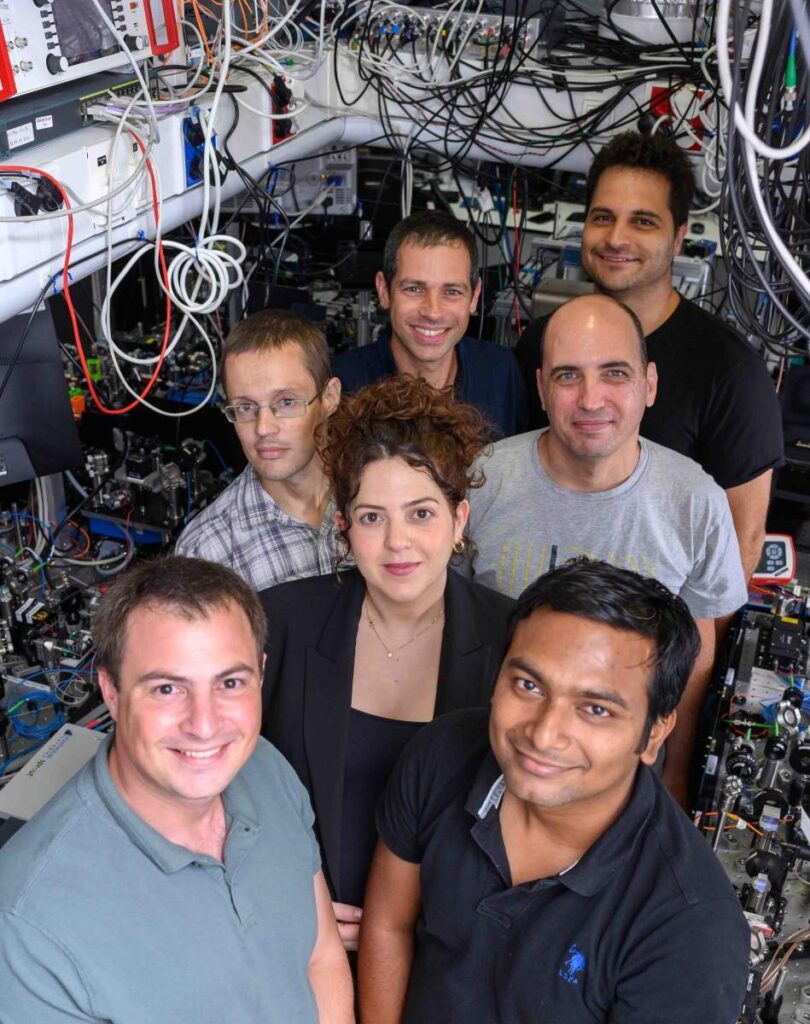
From bottom left, clockwise: Dr Lee Drori, Tomer Danino Zohar, Dr Alexander Poddubny, Professor Ofer Firstenberg, Dr Gal Winer, Dr Eilon Poem and Dr Bankim Chandra Das
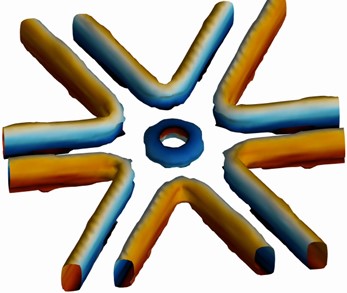
A vortex ring and lines created by the influence of three photons on one another. The colour describes the phase of the electric field, which completes a 360-degree rotation around the vortex core